Abstract
Eco-friendly technologies are of great significance to agricultural sustainability due to the environmental damage caused by agricultural activities. Here, we report a wind and rain energy-driven electrical stimulation system for enhancing crop production. The system is based on an all-weather triboelectric nanogenerator (AW-TENG), which is composed of a bearing-and-hair structured triboelectric nanogenerator (TENG) and a raindrop-driven TENG. Treated by the self-generated high-voltage electric field, the system can increase pea seeds germination speed by ~26.3% and pea yield by ~17.9%. By harvesting environmental wind and raindrop energy, the AW-TENG can be used to drive various agricultural sensors for optimizing plant growth. This work provides a fresh direction for self-powered systems in safe, efficient and eco-friendly agricultural production improvement and may profoundly contribute to the construction of a sustainable economy.
This is a preview of subscription content, access via your institution
Access options
Access Nature and 54 other Nature Portfolio journals
Get Nature+, our best-value online-access subscription
$29.99 / 30 days
cancel any time
Subscribe to this journal
Receive 12 digital issues and online access to articles
$119.00 per year
only $9.92 per issue
Buy this article
- Purchase on Springer Link
- Instant access to full article PDF
Prices may be subject to local taxes which are calculated during checkout





Similar content being viewed by others
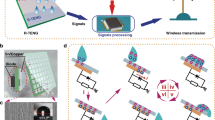
Data availability
All relevant data are included in the article, Supplementary Information and in the Source Data files provided with this paper. All the other raw data are available from the authors on request.
References
Gruber, K. Re-igniting the green revolution with wild crops. Nat. Plants 2, 16048 (2016).
Kesavan, P. C. & Swaminathan, M. S. Modern technologies for sustainable food and nutrition security. Curr. Sci. 115, 1876–1883 (2018).
Duhan, J. S. et al. Nanotechnology: the new perspective in precision agriculture. Biotechnol. Rep. 15, 11–23 (2017).
Molina-Santiago, C. & Matilla, M. A. Chemical fertilization: a short-term solution for plant productivity? Microb. Biotechnol. 13, 1311–1313 (2020).
Landrigan, P. J. et al. The Lancet Commission on pollution and health. Lancet 391, 462–512 (2018).
Schmiedchen, K., Petri, A. K., Driessen, S. & Bailey, W. H. Systematic review of biological effects of exposure to static electric fields. Part II: Invertebrates and plants. Environ. Res. 160, 60–76 (2018).
Krueger, A. P., Kotaka, S. & Andriese, P. C. Studies on the effects of gaseous ions on plant growth. I. The influence of positive and negative air ions on the growth of Avena sativa. J. Gen. Physiol. 45, 879–895 (1962).
Costanzo, E. The influence of an electric field on the growth of soy seedlings. J. Electrostat. 66, 417–420 (2008).
Acosta-Santoyo, G., Herrada, R. A., De Folter, S. & Bustos, E. Stimulation of the germination and growth of different plant species using an electric field treatment with IrO2-Ta2O5|Ti electrodes. J. Chem. Technol. Biotechnol. 93, 1488–1494 (2018).
Fan, F. R., Tian, Z. Q. & Wang, Z. L. Flexible triboelectric generator. Nano Energy 1, 328–334 (2012).
Wu, C., Wang, A. C., Ding, W., Guo, H. & Wang, Z. L. Triboelectric nanogenerator: a foundation of the energy for the new era. Adv. Energy Mater. 9, 1802906 (2019).
Ouyang, H. et al. Symbiotic cardiac pacemaker. Nat. Commun. 10, 1821 (2019).
Song, Y. et al. Wireless battery-free wearable sweat sensor powered by human motion. Sci. Adv. 6, eaay9842 (2020).
Shi, Q. et al. Deep learning enabled smart mats as a scalable floor monitoring system. Nat. Commun. 11, 4609 (2020).
Luo, J., Gao, W. & Wang, Z. L. The triboelectric nanogenerator as an innovative technology toward intelligent sports. Adv. Mater. 33, 2004178 (2021).
Luo, J. et al. Flexible and durable wood-based triboelectric nanogenerators for self-powered sensing in athletic big data analytics. Nat. Commun. 10, 5147 (2019).
Leung, S. F. et al. Blue energy fuels: converting ocean wave energy to carbon-based liquid fuels via CO2 reduction. Energ. Environ. Sci. 13, 1300–1308 (2020).
Jiang, T. et al. Robust swing-structured triboelectric nanogenerator for efficient blue energy harvesting. Adv. Energy Mater. 10, 2000064 (2020).
Li, C. et al. Self-powered electrospinning system driven by a triboelectric nanogenerator. ACS Nano 11, 10439–10445 (2017).
Jiang, C. et al. All-electrospun flexible triboelectric nanogenerator based on metallic MXene nanosheets. Nano Energy 59, 268–276 (2019).
Guo, H. et al. A highly efficient triboelectric negative air ion generator. Nat. Sustain. 4, 147–153 (2021).
Ding, W. et al. Tribopump: a low-cost, hand-powered water disinfection system. Adv. Energy Mater. 9, 1901320 (2019).
Han, K. et al. Self-powered electrocatalytic ammonia synthesis directly from air as driven by dual triboelectric nanogenerators. Energ. Environ. Sci. 13, 2450–2458 (2020).
Shang, W. et al. Rotational pulsed triboelectric nanogenerators integrated with synchronously triggered mechanical switches for high efficiency self-powered systems. Nano Energy 82, 105725 (2021).
Wu, J., Xi, Y. & Shi, Y. Toward wear-resistive, highly durable and high performance triboelectric nanogenerator through interface liquid lubrication. Nano Energy 72, 104659 (2020).
Zhou, L. et al. Simultaneously enhancing power density and durability of sliding-mode triboelectric nanogenerator via interface liquid lubrication. Adv. Energy Mater. 10, 2002920 (2020).
Wang, S., Xie, Y., Niu, S., Lin, L. & Wang, Z. L. Freestanding triboelectric-layer-based nanogenerators for harvesting energy from a moving object or human motion in contact and non-contact modes. Adv. Mater. 26, 2818–2824 (2014).
Chen, P. et al. Rationally segmented triboelectric nanogenerator with a constant direct-current output and low crest factor. Energ. Environ. Sci. 14, 4523–4532 (2021).
Hou, F. F. & Thseng, F. S. Studies on the screening technique for pregermination flooding tolerance in soybean. Jpn. J. Crop. Sci. 61, 447–453 (1992).
Chun, M. S., Ying, Z. H. & Hui, K. X. Effects of dry-heat treatment at 76°C on germination and vigour of radish seed. Seed. Sci. Technol. 31, 193–197 (2003).
Hillis, D. G., Fletcher, J., Solomon, K. R. & Sibley, P. K. Effects of ten antibiotics on seed germination and root elongation in three plant species. Arch. Environ. Con. Tox. 60, 220–232 (2011).
Basiry, M. & Esehaghbeygi, A. Cleaning and charging of seeds with an electrostatic separator. Appl. Eng. Agric. 28, 143–147 (2012).
Wang, G. et al. The effect of high-voltage electrostatic field (HVEF) on aged rice (Oryza sativa l.) seeds vigor and lipid peroxidation of seedlings. J. Electrostat. 67, 759–764 (2009).
Luo, K. et al. Direct exposure of wheat seeds to high-voltage electrostatic fields adversely affects the performance of Sitobion avenae (Hemiptera: Aphididae). J. Econ. Entomol. 109, 2418–2423 (2016).
Wang, J. et al. Investigation of low-current direct stimulation for rehabilitation treatment related to muscle function loss using self-powered teng system. Adv. Sci. 6, 1900149 (2019).
Jiang, D. et al. Emerging implantable energy harvesters and self-powered implantable medical electronics. ACS Nano 14, 6436–6448 (2020).
Wang, X., Li, X. & Li, Y. A modified Coomassie brilliant blue staining method at nanogram sensitivity compatible with proteomic analysis. Biotechnol. Lett. 29, 1599–1603 (2007).
Murr, L. E. Plant growth response in an electrokinetic field. Nature 207, 1177–1178 (1965).
Fanizza, G., Dellagatta, C. & Bagnulo, C. A nondestructive determination of leaf chlorophyll in Vitis vinifera. Ann. Appl. Biol. 119, 203–205 (1991).
Ruiz-Espinoza, F. H. et al. Field evaluation of the relationship between chlorophyll content in basil leaves and a portable chlorophyll meter (SPAD-502) readings. J. Plant Nutr. 33, 423–438 (2010).
Uddling, J., Gelang-Alfredsson, J., Piikki, K. & Pleijel, H. Evaluating the relationship between leaf chlorophyll concentration and SPAD-502 chlorophyll meter readings. Photosynth. Res. 91, 37–46 (2007).
Sui, X. et al. The complex character of photosynthesis in cucumber fruit. J. Exp. Bot. 68, 1625–1637 (2017).
Krupa, S. et al. Ambient ozone and plant health. Plant Dis. 85, 4–12 (2001).
Sandermann, H. Ozone and plant health. Annu. Rev. Phytopathol. 34, 347–366 (1996).
Bortolin, R. C. et al. Effects of chronic elevated ozone concentration on the redox state and fruit yield of red pepper plant Capsicum baccatum. Ecotox. Environ. Safe. 100, 114–121 (2014).
Singh, P., Singh, I. & Shah, K. Reduced activity of nitrate reductase under heavy metal cadmium stress in rice: an in silico answer. Front. Plant. Sci. 9, 1948 (2019).
Lee, S. J. et al. Bacillus subtilis strain L1 promotes nitrate reductase activity in Arabidopsis and elicits enhanced growth performance in Arabidopsis, lettuce, and wheat. J. Plant. Res. 133, 231–244 (2020).
Wong, M. C., Xu, W. & Hao, J. Microplasma-discharge-based nitrogen fixation driven by triboelectric nanogenerator toward self-powered mechano-nitrogenous fertilizer supplier. Adv. Funct. Mater. 29, 1904090 (2019).
Tang, W., Chen, B. D. & Wang, Z. L. Recent progress in power generation from water/liquid droplet interaction with solid surfaces. Adv. Funct. Mater. 29, 1901069 (2019).
Lai, Y. C., Hsiao, Y. C., Wu, H. M. & Wang, Z. L. Waterproof fabric-based multifunctional triboelectric nanogenerator for universally harvesting energy from raindrops, wind, and human motions and as self-powered sensors. Adv. Sci. 6, 1801883 (2019).
Acknowledgements
This research was supported by the National Key R&D Project from Minister of Science and Technology (no. 2016YFA0202704), National Natural Science Foundation of China (no. 52002028), National Science Fund of Excellent Young Scholars of China (grant no. 31922063), China Postdoctoral Science Foundation (no. BX20190324, 2020M680650) and Beijing Municipal Science & Technology Commission (no. Z171100002017017). Experiments on plant phenotypes were supported by Beijing EcoTech Science and Technology Ltd, Ecolab. We also thank S. Dai for helpful assistance in experiments.
Author information
Authors and Affiliations
Contributions
J.L., J.P. and Z.L.W. conceived the idea. X.L., J.L. and K.H. designed and fabricated the device. X.L., J.L., X.S. and Z.R. carried out the pea planting, phenotype characterization and physical and chemical experiments. X.L., J.L. and X.S. performed the electrical measurement and Supplementary Videos. Y.X. and Y.Y. provided assistance with the experiments. X.L., J.L., J.P. and Z.L.W. wrote the manuscript. All the authors discussed the results and commented on the manuscript.
Corresponding authors
Ethics declarations
Competing interests
The authors declare no competing interests.
Peer review information
Nature Food thanks Zhuangzhi Sun, Jianhua Hao and the other, anonymous, reviewer(s) for their contribution to the peer review of this work.
Additional information
Publisher’s note Springer Nature remains neutral with regard to jurisdictional claims in published maps and institutional affiliations.
Supplementary information
Supplementary Information
Supplementary Figs. 1–33 and Notes 1–11.
Supplementary Video 1
The detailed rotation situations of the traditional rotation-mode TENG under the simulated natural wind.
Supplementary Video 2
The detailed rotation situations of the BH-TENG under the simulated natural wind.
Supplementary Video 3
Nitrogen oxides generated by the AW-TENG induced microplasma discharge.
Supplementary Video 4
O3 generated by the AW-TENG induced microplasma discharge.
Supplementary Data 1
Statistical source data of Supplementary figures.
Supplementary Data 2
Statistical source data of Supplementary figures.
Source data
Source Data Fig. 1
Statistical source data.
Source Data Fig. 2
Statistical source data.
Source Data Fig. 3
Statistical source data.
Source Data Fig. 4
Statistical source data.
Source Data Fig. 5
Statistical source data.
Rights and permissions
About this article
Cite this article
Li, X., Luo, J., Han, K. et al. Stimulation of ambient energy generated electric field on crop plant growth. Nat Food 3, 133–142 (2022). https://doi.org/10.1038/s43016-021-00449-9
Received:
Accepted:
Published:
Issue Date:
DOI: https://doi.org/10.1038/s43016-021-00449-9
This article is cited by
-
Structural disruption of melanin-like polymers with boosted UV protection
Science China Chemistry (2024)
-
Triboelectric nanogenerators
Nature Reviews Methods Primers (2023)
-
CNT-PDMS foams as self-powered humidity sensors based on triboelectric nanogenerators driven by finger tapping
Scientific Reports (2023)
-
Recent advances in high-performance triboelectric nanogenerators
Nano Research (2023)
-
Multidiscipline Applications of Triboelectric Nanogenerators for the Intelligent Era of Internet of Things
Nano-Micro Letters (2023)